COMPUTATIONAL GENOMICS
THE "HOW" OF SCIENCE

GENE EXPRESSION
WHAT IS GENE EXPRESSION?
You know that gene expression is the process where the information that the DNA base pairs contain is made into a functional product, like a protein or RNA that serves a specific purpose in the cell. But how do we figure out what each piece of DNA codes for? There are several techniques that scientists use to try to assign specific functions to segments of DNA.

This diagram shows the complex system of how the genes in the nucleus of the cell are expressed to create proteins [1]
Scientists know that a mutation in a specific gene can be a contributing factor in the causation of a disease. However, since a gene contains thousands of places where a mutation can occur, it can difficult to pinpoint where exactly the mutation occurred.
A DNA microarray is a technique that scientists use to determine the expression of certain genes and whether they contain mutations. The microarray is a microscopic plate printed with dots containing the known sequences of DNA in specific places on the plate [1].
To determine whether an individual's DNA contains the mutation that could be expressed as a disease, the scientists need to obtain a sample of the individual's DNA, as well as a control sample that is known not to contain the mutation being tested for. Next, the scientists use a fluorescent dye to mark both the control and the experimental sample (green for the experimental sample and red for the control).
The samples are then allowed to bind to the DNA on the microarray. If the experimental sample contains the mutation, it will not be able to bind to the corresponding spots on the microarray. Since only the control will be able to bind to the microarray, the spot will turn red. However, if the DNA does not contain the mutation being tested for, both samples will be able to bind to the spot on the plate and the mixture of both green and red samples will turn the spot yellow [1][2].
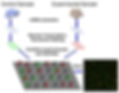
This diagram depicts the process of microarray technology [2]
This image shows how the samples that are being analyzed are loaded into a microarray plate during the process of microarray technology [3]
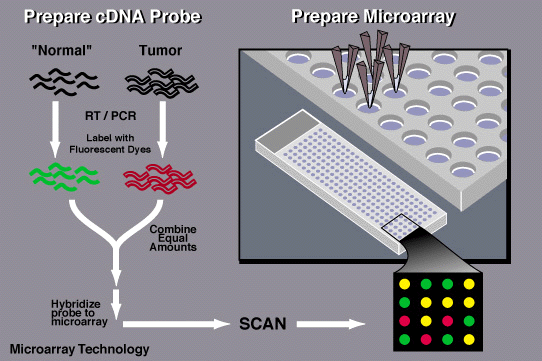
MICROARRAY TECHNOLOGY
APPLICATIONS OF MICROARRAYS
Gene Discovery: Scientists can use Microarray technology to study the functions of new genes and gain a deeper understanding of their unique functions. Using Microarrays, scientists are able to see how genes are expressed in a variety of environments.
Disease Diagnosis: Microarray technology helps scientists gain a deeper understanding of the causes and distinct behavior of different diseases. Researchers are able to use the technology to classify types of cancer based on an understanding of the patterns of gene activity in the tumors. With more a specific and extensive understanding of the tumor behavior, pharmaceutical companies can develop drugs that more accurately address the specific characteristics of the disease [3].
Drug Discovery: Microarray technology is applicable in discovering new drugs that can accurately combat the effects of proteins created by damaged and diseased cells. By studying the genetic makeup of diseased cells, scientists gain a better understanding of the expression of damaged genes and are able to create drugs to reverse those effects.
Toxicological Research: Scientists are able to use microarrays to study the effects that various toxins can have on the genetic makeup of cells. Using microarrays, scientists study how the genetic profile of a cell is altered by the introduction of a toxin [3].


This image summarizes how microarrays are used in disease diagnosis and gene correlation studies [5]
The infographic above shows the various applications where microarrays can be utilized [4].
EXPRESSED SEQUENCE TAGS (ESTS)
ESTs are small DNA sequences that represent genes expressed in certain cells or tissues in different organisms. Scientists use these bits of DNA to find complementary strands in a portion of chromosomal DNA. The idea is that the part of the chromosome that codes for the same proteins as the EST sample will bind to the sample, making the process of identifying those genes in the large chromosome more efficient [4].
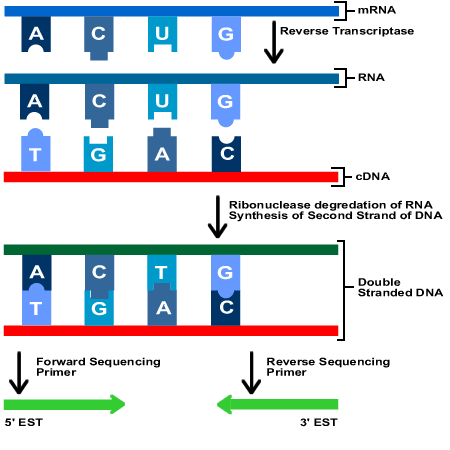
This picture summarizes the process of converting DNA into forward and reverse ESTs [7]
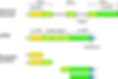
This diagram shows how the genomic structure of DNA is broken into two types of individual ESTs [6]
DNA consists of many non-coding regions of sequences, making identification of genes difficult. In order to create a strand of DNA without these non-coding regions, or introns, the gene must be converted into messenger RNA (mRNA). mRNA serves a template for the protein synthesis process and does not contain any introns. Due to this, using mRNA is crucial to identifying genes in the large expanse of the human genome.
However, mRNA is very unstable outside the cell and needs to be converted into cDNA to be useful in the identification process. Complementary DNA or cDNA is perfect for this process because it does not contain any introns (unlike the original DNA) and can be handled outside the cell (unlike mRNA).
After being successfully converted into complementary DNA by an enzyme, the cDNA is sequenced into two kinds of ESTs, 3' ESTs and 5' ESTs. The 5' EST is obtained from the beginning portion of cDNA, which normally codes for the protein and is similar throughout a gene family. The 3' EST is sequenced from the end of the DNA and normally contains untranslated regions (or regions of DNA that don't specifically code for a protein) [4][5].
ESTs and Genome Maps
Scientists use ESTs to generate genome maps that can help them quickly find specific genes that they are looking for. Currently, the most popular genome mapping technique is using STS (or sequenced tag sites). An STS is a short sequence of DNA that only appears once throughout a genome. 3'ESTs are especially helpful in finding these STS to create genome maps.
ESTs as Gene Discovery Resources
ESTs are valuable tools in searching for correlations between genes and diseases because they can identify the coding parts of the DNA with extreme efficiency. Scientists are able to use ESTs as a kind of homing device to find specific sequences of DNA that they can then analyze to determine if it contains mutations.
Using ESTs, scientists have been able to isolate the genes that may contribute to the causation of Alzheimer's, colon cancer, and many other diseases [4].
WHAT ARE ESTs USED FOR?
SERIAL ANALYSIS OF GENE EXPRESSION (SAGE)
Serial analysis of gene expression, or SAGE, is an experimental technique that scientists use to produce a snapshot of mRNA in a sample population. The SAGE method isolates unique sequence tags of mRNA and links them into a large DNA molecule for sequencing [6] [7].

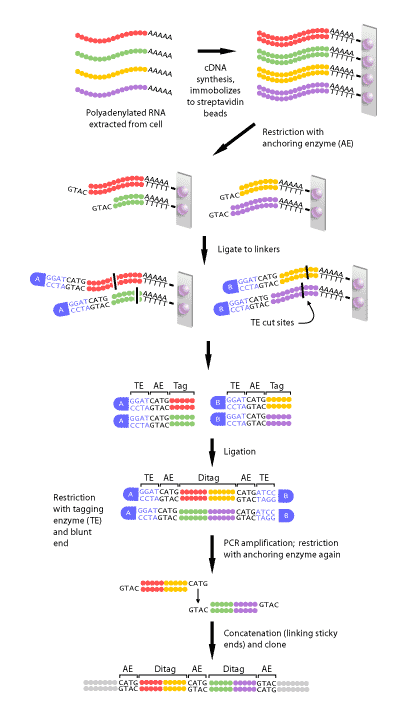
This diagram depicts the process of using cDNA and a concatemer to analyze the expression of genes [8]
First, the mRNA in the sample is converted to cDNA and purified.
Step 1: mRNA is isolated and reverse transcription and biotinylated primers are used to generate the corresponding cDNA for the sequence.
Step 2: The biotin from the primers that were added in the previous step interact and bind with streptavidin beads.
Step 3: Next, the cDNA is cut using an endonuclease restriction enzyme. The restriction enzyme will cut the cDNA into varying lengths based on where the restriction site is on each fragment. Now, the streptavidin beads are
bound to various cDNA fragments, all with different lengths but the same DNA sequence at their exposed end.
Step 4: Any cut cDNA that isn't bonded to a bead is rinsed away, leaving only the fragments that are specifically needed. The solution is now split into two samples.
Second, the cDNA is tagged:
: Next, an oligonucleotide – either A or B – with a sticky end, a recognition site for a tagging enzyme, and unique primer sequence is added to each solution. These oligonucleotides Step 5attach to the cDNA.
Step 6: A tagging enzyme is used to further cut the cDNA. The tagging enzyme recognizes the site from the oligonucleotide (added in step 5) and separates the cDNA from the beads. This leaves a strand of about 15 nucleotides.
Step 7: The cut cDNA fragments are now repaired using DNA polymerase. This produces blunt ends at the exposed end of the cDNA fragments.
This graphic shows what the extracted RNA samples look like throughout the process of SAGE. [9]
Third, the cDNA fragments are connected:
Step 8: The blunt ends of the fragments are connected leaving the A and B adaptors (attached during step 5) on either end. Using the specific primers (also added during step 5) PCR is performed to amplify the newly connected fragment. This new, larger fragment is called a ditag.
Step 9: An anchoring enzyme is used again to cut away the A and B oligonucleotides. This allows the ditags to connect and form longer chains of cDNA (we call this cDNA concatemers). Each individual ditag is separated in the chain by its specific anchoring enzyme recognition site.
Lastly, the concatemers are replicated and sequenced:
Step 10: The concatemers are transformed into bacteria that are allowed to replicate the cDNA.
Step 11: The SAGE process is complete and the cDNA can now be sequenced and analyzed [8].
HOW IS SAGE USED?
-
SAGE has been used to analyze how genes are expressed in a cancerous cell in comparison to a healthy one
-
Scientists use SAGE to study the different transcripts in each cell
-
SAGE provides an accurate and efficient comparison between the expression of genes in both diseased and healthy cells.
-
Discover different genes and investigate how they are expressed [6].
MASSIVELY PARALLEL SIGNATURE SEQUENCING
Massively parallel signature sequencing is a technique similar to SAGE used to quantify and analyze genetic sequences [9].
MPSS uses very similar techniques as SAGE: The mRNA is converted into cDNA strands. The cDNA strands are bonded with an oligonucleotide tag. Fluorescent-labeled probes are used to produce a signature sequence of 16-20 base pairs, which is used to identify each fragment (This step diverges from the methods used in SAGE). Fluorescent imaging captures a parallel image of the DNA by interpreting the signal from all of the beads [9].

This picture shows the process of preparing and tagging a DNA sample in order to analyze its function using MPSS [9].
HOW IS MPSS DIFFERENT FROM SAGE?
While MPSS and SAGE techniques for sequencing DNA are very similar in their methods, there are some significant advantages to using MPSS. For example, MPSS uses longer tags (usually around 16-20 bp) than the 9-10 bp that are used by SAGE technology. MPSS has a significantly larger library of sequences than SAGE, which usually contains 20 times less information than the MPSS library.

This graph contrasts the library size of two similar gene expression techniques- SAGE and MPSS [10].
However, there are some significant disadvantages for MPSS such as the fact that MPSS can lose information, due to a lack of restriction enzyme recognition sites and ambiguity in the annotation process. If you are looking for specificity and sensitivity, MPSS is clearly a more valuable option. However, the technology is very limited in availability since it is only offered through Lynxgen Therapeutics, Inc [9].